In the last post, I gave a broad overview of both traditional and modern usage of the Fly Agaric mushroom as a visionary drug, but also as a mild stimulant and party drug. I also briefly discussed the major active components, muscimol and ibotenic acid and, importantly, that muscimol can be directly derived from ibotenic acid by removal of a carbon dioxide molecule (decarboxylation). In this second post, I want to discuss in much more detail the chemistry and pharmacology of both ibotenic acid and muscimol and the possible mechanisms underlying their psychoactive effects.
Although muscimol is generally considered to be the important psychoactive component of Amanita muscaria, there remains some disagreement about whether ibotenic acid has any positive role in its effects. Both muscimol and ibotenic acid are highly water soluble, but can pass through the blood brain barrier using protein transporters for amino acids. So, although it’s plausible that both could contribute to the mushroom’s psychoactive effects, much of the ibotenic acid is decarboxylated to muscimol in the liver, so it’s not clear how much actually makes it into the brain unaltered. Human studies are extremely limited, but consumption of pure ibotenic acid doesn’t appear to be psychedelic: nausea, muscle twitching, facial flushing and other peripheral effects (i.e. outside the brain) are usually followed by drowsiness and then sleep. Muscimol also produces similar peripheral effects, but these are usually accompanied by the more desirable central effects (i.e. inside the brain), such as drunkenness, stimulation, and alterations in visual and sometimes auditory and tactile perception. Rarely, however, do these effects approach anything resembling those of LSD or psilocybin, and confusion and disorientation are also often pronounced. So, despite the limited human data available, we can be fairly confident that muscimol is responsible for the more visionary effects of the Fly Agaric, albeit straying far from a clean psychedelic experience for most people.
It’s also often pointed out that ibotenic acid is neurotoxic and used to chemically lesion the brains of experimental animals. Whilst this is true, it certainly doesn’t mean that ingesting Fly Agaric is likely to cause any kind of damage to the brain. In experimental studies, ibotenic acid is applied in high concentrations directly into neural tissue, which obviously isn’t the case with oral consumption. So, whilst the likelihood of brain damage is probably overstated, we can at least say that, on balance of evidence, ibotenic acid is more likely to contribute to negative than visionary effects after consuming the mushroom. As such, it makes sense to attempt to decarboxylate the ibotenic acid to muscimol prior to ingestion. Modern Fly Agaric users usually call for the mushroom either to be dried under a moderate heat or boiled in slightly acidic water to achieve this.
Drying the mushrooms outside under the sun for three days will reduce the ibotenic acid by about 50%, with muscimol increasing over 10-fold. Increasing the sunning time to 10 days or longer will bring the ibotenic acid down to less than 10% of its initial level, although longer periods of sun exposure also cause some loss of muscimol, either by some kind of direct oxidative degradation or by diversion of ibotenic acid into an alternative UV-mediated conversion to muscazone. Since there’s practically no data on the human psychoactive effects or toxicity of muscazone, it’s probably best to avoid drying under the sun — a similar rate of conversion, with less risk of accumulating muscazone, can be achieved by drying the mushrooms beside a heater inside the home.
The degree of ibotenic acid decarboxylation increases with temperature, although above 80c muscimol also begins to rapidly degrade. Drying in an oven kept at 60-70c would thus seem to be optimal for rapid and efficient decarboxylation whilst minimising loss of muscimol.
In boiling water, the rate of ibotenic acid decarboxylation increases as the acidity of the water is increased. At pH4, around 90% of the ibotenic acid will be decarboxylated to muscimol after boiling for around an hour. Of course, since both ibotenic acid and muscimol are highly water soluble, the mushrooms themselves will be completely inactive by this point, and the water itself should be consumed (use lemon juice or citric acid to adjust the pH).
Once they get into the brain, ibotenic acid and muscimol exert opposing effects on neurons. Ibotenic acid is excitatory, meaning it activates neurons — it does this by binding and activating a type of glutamate receptor called the NMDA receptor, allowing positively-charged ions to flow into the neuron. See my post on the pharmacology of ketamine for more details on the NMDA receptor:
Muscimol, on the other hand, binds and activates the major inhibitory receptors in the brain, the GABA receptors, which are used to suppress neurons and control the balance of excitatory vs inhibitory activity. The endogenous neurotransmitter, GABA, is (obviously) the natural agonist of the GABA receptor and, similar to the NMDA receptor, binding of GABA causes the receptor’s ion channel to open. However, unlike the NMDA receptor which allows positively-charged ions to flow into the neuron, the GABA channel selectively gates the flow of negatively-charged chloride ions. These make the inside of the neuron more negative — hyperpolarisation — and pull its membrane potential further from the firing threshold, rendering the neuron less excitable and less likely to fire.
A large range of molecules can interact with the GABA receptor and alter the way it behaves. Ethanol, for example, activates GABA receptors and causes a general suppression of cortical activity. This explains many of the effects of this most popular of psychoactive agents: a feeling of relaxation and decreased anxiety, loss of inhibitions and increased sociability, followed by outright drunkenness as the night progresses and, ultimately, drowsiness and sleep. Incidentally, the cerebellum, the small highly-folded “mini-brain” sitting just beneath and at the back of your cortex, is responsible for the fine-tuning of movement, including walking and speech. It’s also highly sensitive to ethanol, which is why you might begin to slur your speech or have trouble pacing a straight line after a few too many single malts.
Muscimol also activates GABA receptors, so the drowsiness and general drunkenness experienced after consuming Fly Agaric is quite simple to explain. The more visionary effects much less so, but we can obtain some clues by looking at the chemical pharmacology of the GABA receptors and the molecules that modulate their activity in more detail.
Many of the most popular and well-known drugs used as sedatives to treat insomnia and anxiety are also GABA activators. These include the benzodiazepines, such as diazepam (first marketed as Valium) and alprazolam (Xanax), and the newer Z-drugs: zaleplon, zolpidem (Ambien), zopiclone, and eszopiclone. Interestingly, hallucinations are a rare, but well-described, side-effect of the Z-drugs, particularly at high doses. The benzodiazepines, however, are not usually associated with hallucinations, and neither is alcohol (not counting alcohol withdrawal syndromes).
So, why should certain GABA-activating molecules elicit hallucinatory effects, whilst others fail to do so? In fact, why should molecules that generate cortical inhibition be psychedelic at all? Well, it turns out, as is so often the case in neuropharmacology, that “GABA receptor activation” is a little more complicated than we once thought.
Firstly, there are broadly two types of GABA receptor: GABA-A and GABA-B. GABA-A receptors are the ligand-gated ion channels we’re interested in here. The GABA-B receptors are G-protein coupled receptors, much like the serotonin (5HT) receptors. We’ll say nothing more of them here.
All GABA-A receptors are built from five protein subunits that combine to form the complete receptor ion channel.
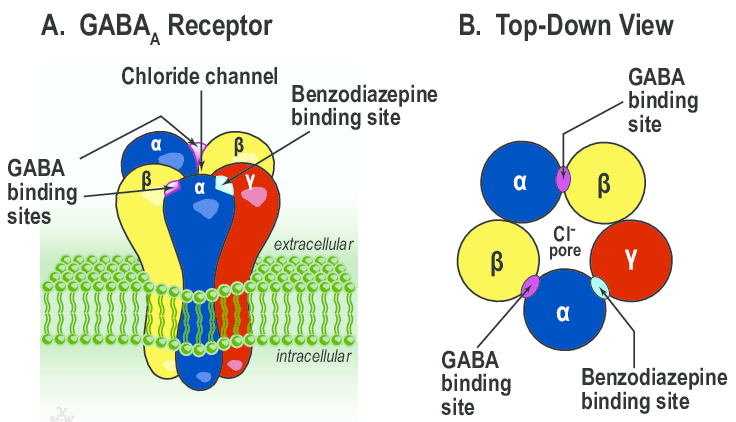
However, there are at least 19 different types of these subunits separated into 8 subtypes and named using the Latin alphabet from alpha to theta, as well as a numerical subscript when there’s more than one member of a subtype:
Six alpha (α1-6), three beta (β1-3), three gamma (γ1-3), three rho (ρ1-3), and one each of the delta (δ), epsilon (ε), pi (π), and theta (θ).
Different combinations of these subunit types can generate a large range of different receptor isoforms (an isoform is just a particular combination of five subunits). Most GABA-A receptors are built from two α, two β, and one γ subunit (arranged clockwise α β α β γ), although a δ, ε, π, or θ subunit can replace the γ in different isoforms. The subunit composition of the receptor can affect its behaviour, including how long the chloride channel remains open and, crucially, which molecules will bind and modulate its behaviour.
GABA-acting drugs will often bind most strongly to particular subunit types, making them more or less selective for receptor isoforms that contain those subunits. Furthermore, different regions of the brain will often tend to express different GABA-A receptor isoforms, meaning such drugs will also tend to be selectively active in those areas of the brain. For example, the benzodiazepines, such as diazepam, bind strongly to the α 1, 2, 3, and 5 subtypes (as long as a γ subunit is also present, since they bind at the α-γ interface). The Z-drugs, however, are more selective for different α-subunit subtypes. Zolpidem, better known as Ambien, for example, binds strongly and selectively to the α1 subunit, which is thought to be responsible for its efficacy at promoting sleep.
Muscimol also only binds strongly to a subset of GABA-A receptor isoforms — most likely those containing the α4 and δ subunits — and accumulates in those areas of the brain that express those isoforms, including the thalamus and hippocampus. It’s likely that this unusual binding profile accounts for the psychedelic effects of muscimol not seen with other GABA-activating drugs, such as the benzodiazepines. Hamilton Morris reliably informs me that the sedative gaboxadol (a GABA-activating derivative of muscimol) is also psychedelic at high doses. Having just landed in Japan from the US and attempting to outwit his disrupted circadian rhythm, he tried to “knock himself out” by taking a particularly heavy dose. However, instead of passing out cold, he unintentionally had “one of the most intense psychedelic experiences of my life.” Gaboxadol, like muscimol, selectively binds and activates a particular GABA-A receptor isoform highly expressed in the thalamus.
Our 2-hour conversation on psychedelic neuroscience is available (to his Patreon subscribers) here:
https://www.patreon.com/posts/pod-65-dr-andrew-76525101
So, since different drugs will tend to suppress activity in the particular areas of the brain expressing the GABA-A isoforms to which they bind, it’s somewhat simplistic to assume that a molecule that activates GABA receptors (and thus inhibits neurons) will cause a global reduction of neural activity across the entire brain. Furthermore, it’s also possible for GABA-A activating molecules to actually increase activity in certain regions of the brain, since cortical areas are often charged with regulating the activity of other areas. The claustrum, for example, maintains inhibitory control over the activity of large regions of the cortex. Electrically stimulating the claustrum will briefly cause unconsciousness by strongly increasing its suppression of cortical activity, whereas inhibiting the claustrum has the opposite effect: Salvinorin (which is extremely psychedelic) also works by activating inhibitory receptors — the kappa opioid receptors that inhibit the claustrum, releasing the cortex from its control. See my earlier post on salvinorin for further details:
More generally, the overall balance of excitation (e.g. by glutamate) and inhibition (e.g. by GABA) in the brain (the “E/I balance”) is of critical importance in processing sensory information, constructing and maintaining the world model, and establishing synchronous activity across large areas of the cortex. Disruption of these processes also underlies the psychedelic effects of the classic 5HT2A agonists (LSD, psilocin, etc). And, perhaps tellingly, unusual patterns of GABA receptor expression are also seen in schizophrenic patients, particularly in those areas associated with hallucinations: the temporal lobes at the sides of the cortex.
So, taken together, the picture becomes rather complex: muscimol disturbs the E/I balance in specific areas of the brain (by activating only a subset of GABA-A receptor isoforms), disrupting important sensory processing and world model construction processes, as well as potentially releasing other areas from their control. The thalamus, for example, has crucial roles in gating the flow of sensory information into the cortex, as well as regulating the flow of information between its areas and synchronising their activity. It’s reasonable to surmise that disruption of both thalamic and temporal lobe activity by muscimol or gaboxadol might be at least partially responsible for their visionary effects. But the complete picture remains far from complete and somewhat out of focus. Unfortunately, since Psilocybe mushrooms are currently very much centre stage in psychedelic research, there isn’t too much motivation to study mechanistic underpinning of Amanita muscaria’s psychedelic effects in humans. Perhaps that oversight will be remedied in the future — there’s certainly still a lot we don’t know about this mushroom’s fascinating chemistry and pharmacology.
Love this post! I’ve heard muscimol classified as a depressant, psychedelic and a dissociative. Is it possible it can act as all three at different concentrations in the brain? Or is it the case that anything that alters ones view of the world is a psychedelic?