Do Different Psilocybe Mushrooms Produce Different Effects? — Part 2
Do norbaeocystin, baeocystin, and aeruginascin enter the brain?
In the previous post, I discussed how a recent analysis of 30 psychedelic mushroom species revealed large variations the levels of five major tryptophan-derived alkaloids, both between species and within samples of the same species.
See Part I here:
It’s perfectly reasonable to surmise that the unique proportions of the five major psychoactive tryptamines in a particular species/strain of Psilocybe mushroom could elicit effects characteristic of that species/strain. However, this is only likely to be the case if the different tryptamines are experientially distinct or, through differential receptor binding profiles, are able to modulate the effects of the other alkaloids. It’s also possible that other minor components — such as the beta-carbolines — could modulate the psychedelic experience, but I’ll discuss this in Part 3 (the final part of this short series).
Although it’s very difficult to predict the experiential effects of a mixture of several different psychoactive drugs, one thing we can say for sure: If a molecule is unable pass from the blood into the brain, no matter how strongly it binds to a particular receptor, it will not be psychoactive. So, whether or not the tryptamines in Psilocybe mushrooms can enter the brain is of central importance when considering any kind of entourage effect, and is what I will focus on in this post.
Psilocybin is the major tryptamine alkaloid in most Psilocybe mushrooms but is not the active component. Psilocybin is a pro-drug metabolised to the active form, psilocin, by alkaline phosphatases in the GI tract. Only the dephosphorylated psilocin form can enter the brain. Norbaeocystin, baeocystin, and aeruginascin are also phosphorylated at the indole 4-position and we can assume that this phosphate is cleaved in the gut.
To gain entry into the brain, and thus to exhibit psychoactive effects, a drug must pass the blood brain barrier (BBB), a highly selective semipermeable layer of cells that prevents molecules in the blood from non-selectively crossing into the brain. In general, the BBB allows non-polar (fat-soluble) molecules to diffuse through — this is important to allow oxygen, carbon dioxide, and hormones to enter and leave the brain. Polar (water soluble) molecules are unable to cross the BBB without specialised transporter proteins (for amino acids, ions, and other molecules important for neural function).
To appreciate how this relates to the tryptamines, let’s first look at serotonin — 5-hydroxy-tryptamine — an important neuromodulator that does not cross the BBB (serotonin used by the brain is manufactured inside the brain, not in the gut).
Serotonin contains two important groups: the hydroxyl (OH) group at the 5-position on the indole ring and the aminoethyl side chain (-CH2CH2NH2). Both hydroxyls and amines are polar groups — they make the molecule more water soluble. As such, serotonin is quite polar overall and cannot diffuse across the BBB.
However, it’s possible to mask the polarity of these groups by adding non-polar moieties, such as methyl (CH3) groups. For example, bufotenine (5-OH-N,N-DMT) differs from serotonin only in sporting two methyl groups on the side chain amine, which masks its polarity and renders the molecule slightly less polar overall. This gives it a better chance at crossing the BBB, although this remains hindered by the polar 5-hydroxyl. Despite having high efficacy at the 5HT2A receptor, bufotenine requires quite high doses to achieve psychedelic effects. Ethnobotanist Jonathan Ott reported modest visionary effects — “colored patterns with eyes closed; visible, albeit fainter, with eyes opened in low light” — above 40 mg (by nasal insufflation) (link). Bufotenine users tend to report quite strong bodily effects, including facial flushing, breathing restriction, and nausea, indicating a largely peripheral action. Animal studies also show that bufotenine is distributed mainly to the heart, lungs, and liver, but to a much lesser extent to the brain, since its ability to cross the BBB is quite weak (link).
Now, if we add a methyl group to the 5-hydroxyl of bufotenine, we get 5-MeO-DMT, a highly potent psychedelic with active doses in the low milligram range (5-10mg). The polarity of the hydroxyl is now masked by the methyl group allowing the molecule to readily cross the BBB. Note that N,N,-DMT, which doesn’t contain that pesky hydroxyl group in the first place, is also quite non-polar and rapidly enters the brain to achieve its astonishing reality-switching effects.
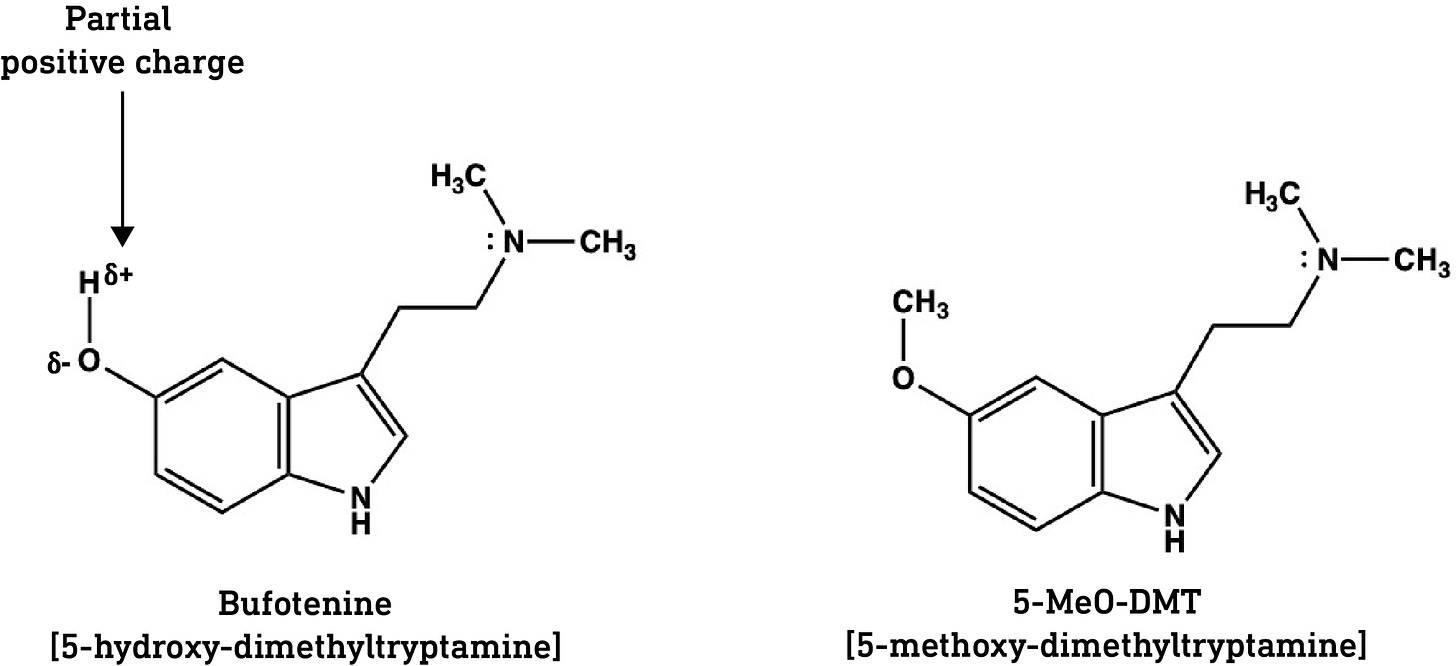
So, what about psilocybin and psilocin? This is where the fun begins…
Psilocybin, as mentioned before, is phosphorylated at the 4-position on the indole — with this highly polar phosphate group, it certainly can’t cross the BBB — but is rapidly dephosphorylated yield psilocin.
At first glance, psilocin — 4-hydroxy-DMT — is simply bufotenine with the hydroxyl group shifted one carbon along the indole ring. And, yet, their pharmacological properties are quite distinct. Firstly, unlike bufotenine, psilocin resists metabolism by monoamine oxidase (MAO) and is active orally. Secondly, psilocin is much better at crossing the BBB than bufotenine…
Why? Shouldn’t that polar hydroxyl make access to the brain difficult?
Well, it turns out that this shift in the hydroxyl position on the indole ring is of critical importance.
The hydrogen atom on the indole hydroxyl carries a partial positive charge and the aminoethyl nitrogen carries a lone pair of electrons (a pair of electrons not involved in covalent bonding), which are, of course, negatively charged. Shifting the hydroxyl one carbon along the ring brings this hydrogen and the lone pair of electrons into an optimal position to form an intramolecular hydrogen bond (a type of relatively weak bond formed between hydrogen and another atom containing a partial negative charge, usually oxygen or nitrogen). Formation of this hydrogen bond masks the polarity of both the hydroxyl and the amine nitrogen, rendering the entire molecule much less polar overall and able to easily cross the BBB.
In addition, the hydrogen-bonded form of the molecule is much more resistant to MAO inactivation — the MAO enzyme struggles to “get hold” of the molecule in this form — rendering psilocin orally active as well. Take a moment and appreciate how beautiful this is.
Does this mean that the dephosphorylated forms of baeocystin and norbaeocystin can also form this hydrogen bond and thus potentially cross the BBB?
Although it isn’t possible to directly visualise this all-important hydrogen bond being formed when psilocin is in solution, its presence can be inferred by measuring how the hydroxyl hydrogen atom behaves in a powerful magnetic field. Proton nuclear magnetic resonance spectroscopy (proton-NMR) gives us a picture of all the hydrogen atoms in a molecule and their electronic environment (a hydrogen atom is a single proton orbited by a single electron).
The frequency at which a proton appears in an NMR spectrum tells us about the density of the electron cloud around the proton, since electrons around a proton shield it to some extent from an applied magnetic field. A more shielded proton will appear at a lower frequency in the NMR spectrum. When a hydrogen is part of a chemical bond — including a hydrogen bond — it donates some of its electron cloud to the bond and its proton experiences more of the applied field (we say that the proton is deshielded) and it shifts to a higher frequency.
In short, it’s possible to tell whether a particular hydrogen is part of a hydrogen bond or not by measuring the frequency of its signal in the proton-NMR spectrum of the molecule. The hydroxyl proton in psilocin appears at 12ppm (ppm, parts per million, is just a measure of frequency/energy), whereas the proton in bufotenine appears at 7.5ppm. This shows that the psilocin hydroxyl proton is more deshielded than that of bufotenine, and thus that the hydroxyl forms a hydrogen bond with the amine in psilocin but not in bufotenine (there are other spectroscopic details used to deduce this, but this is the key evidence) (link).
Crucially, the hydroxyl protons in the dephosphorylated forms of baeocystin and norbaeocystin appear at about the same frequency as psilocin, indicating that they’re also capable of forming the hydrogen bond that masks the hydroxyl and amine polarity. This suggests that all three might readily cross the BBB.
One caveat here is that the two methyl groups in psilocin also help to mask the amine’s polarity. Since there are only one or two methyl groups on the dephosphorylated forms of baeocystin and norbaeocystin, respectively, it’s likely that they are slightly more polar and less able to cross the BBB compared to psilocin. However, it’s worth noting that a primary amine group (with no methyl or other groups attached, as with norbaeocystin) isn’t necessarily a barrier to crossing the BBB, since 5-MeO-aMT is orally active in the sub-10mg range, perhaps suggesting that the polarity of the indole hydroxyl is more important than the amine in restricting access to the brain.
So, on balance of the evidence, thanks to the intramolecular hydrogen bond, it’s perfectly plausible that the dephosphorylated forms of both baeocystin and norbaeocystin might be both resistant to MAO-mediated degradation and sufficiently non-polar to cross the BBB and thus enter the brain. Only then do their receptor binding profiles and possible psychoactivity become relevant.
The locus of action of the classic psychedelics, and often by which they are defined as such, is the 5HT2A serotonin receptor. All classic psychedelics bind and activate this receptor. However, they aren’t perfectly specific. The acute effects of psilocybin, for example, involve at least three serotonin receptors to some degree: 5-HT2A, 5-HT2C and 5-HT1A (link).
4-OH-MT (from baeocystin) binds and activates the crucial 5HT2A receptor, but likely also activates other serotonin receptor subtypes to varying degrees, which might affect its activity (link). DMT, 5-MeO-DMT, and LSD, for example, all act primarily at the 5HT2A receptor, but each activates the receptor in its own unique manner (this is known as functional selectivity and is a topic for another post), as well as acting at different serotonin receptor subtypes to different degrees. As such, they all exhibit quite distinct psychedelic effects. It’s reasonable to hypothesise then that baeocystin (and norbaeocystin) might be experientially different to psilocin, contributing to an overall distinct effect of a particular mushroom species, given that these three alkaloids are often present in different relative proportions (as discussed in Part 1).
Of course, none of this can assure us definitively that either baeocystin and/or norbaeocystin are psychoactive and can thus contribute to an entourage effect. What we need are studies in humans that demonstrate or refute this. Unfortunately, such studies are lacking. Mycologist Jochen Gartz reported a “gentle hallucinogenic experience” after ingesting 4mg of baeocystin (link) and “10 mg of baeocystin were found to be about as psychoactive as a similar amount of psilocybin”, but his results have never been formally repeated in humans. Discouragingly, baeocystin failed to induce the head-twitch response — a well-established indicator of 5HT2A receptor stimulation — in mice, suggesting a lack of psychedelic activity (link).
Watch the head of the mouse on the left after 1mg/kg injection with psilocybin:
However, this animal data doesn’t conclusively prove a lack of psychedelic effects in humans. Furthermore, it’s possible that, whilst being itself non-psychedelic, (nor)baeocystin could modulate the psychedelic effects of psilocin, owing to its differential activity at serotonin receptor subtypes.
So, aside from the rather fascinating chemistry of the 4-hydroxy-tryptamines and Gartz’s self-experimentation, there’s no clear evidence that either baeocystin or norbaeocystin are actually psychoactive in humans. Nor is there conclusive evidence to the contrary. The jury, as they say, is still very much out.
This leaves us then with aeruginascin. The dephosphorylated form — the only plausibly active form — 4-hydroxy-N,N,N-trimethyltryptamine (4-HO-TMT), binds to 5HT1A, 5-HT2B and, most importantly, 5HT2A receptors (link). Unfortunately, 4-HO-TMT is a quaternary amine, meaning the amine group forms four covalent bonds, including three methyl groups. Since this requires usage of the electron lone pair, forming a fourth bond gives the amine nitrogen a positive charge, making the entire molecule highly polar. This pretty much guarantees it cannot pass the BBB, unless it happens to be a substrate for a transporter protein, but I’ve seen no evidence for this.
It has been suggested that a quaternary amine doesn’t necessarily preclude entry into the brain, since the opioid methylnaltrexone, which also contains a charged quaternary amine, produces effects in mice that are usually induced by stimulation of receptors secured behind the BBB (link). However, again, this evidence is far from conclusive and whether or not it has any bearing on aeruginascin’s putative psychoactivity is completely unknown. We should also point out that only Inocybe aeruginascens contains significant levels of aeruginascin, so it’s rather less likely to contribute to an entourage effect than either baeocystin or norbaeocystin in other species.
In summary then, any entourage effect exhibited by different species of Psilocybe (and related) mushrooms is most likely due to varying concentrations of baeocystin and/or norbaeocystin. The chemical evidence suggests that either or both the active forms of baeocystin and norbaeocystin might survive long enough in the bloodstream (escaping MAO degradation) after oral ingestion to reach the brain and passage through the BBB might be possible. On the other hand, animal studies have failed to indicate a psychedelic effect using well-established protocols, but this neither completely rules out psychedelic effects in humans nor a potentially modulatory effect on psilocin.
In Part 3, I’ll discuss the beta-carbolines, the MAO inhibitory tryptamines that have also been detected in Psilocybe mushrooms, as well as other possible alkaloid and non-alkaloid components, and whether or not they might modulate the psychedelic state.
Are you writing these posts specifically for me? Because, this is exactly the content I have been looking for. Thanks so much for putting the effort into these. Excellent work.
Really terrific article that pairs well with my current read of Reality Switch Technologies. Thank you!